The reason for this scarcity is that the synthesis of cannabinochromic acid (CBCA), a precursor of CBC, is inefficient in the cannabis plant, and the biosynthetic pathway of CBCA faces a number of limitations, including the insufficient supply of precursor molecules such as isoprene pyrophosphate and 2, 4-dihydroxy-6-amylbenzoic acid.
In the field of modern medicine, nerve regeneration is one of the hot research topics.
Cannabinochromene (CBC), as a non-psychoactive cannabinoid, is well known for its ability to promote the survival and differentiation of neural stem cells.
However, the amount of CBC in nature is extremely low, and its proportion only accounts for 0.01% to 0.06% of the total product of the cannabis plant, which is much lower than tetrahydrocannabinol (THC) and cannabidiol (CBD).
And the restriction of CBCA synthetase (CBCAS) activity.
In addition, the cannabis plant has a growth cycle of up to 120 days, further increasing the time and economic cost of extracting CBC from the plant.
Aiming at the target of CBCA, the team of Kang Wei and Xue Chuong from Dalian University of Technology used Saccharomyces cerevisiae as a production platform to successfully construct a new biosynthesis path, which not only significantly improved the yield of CBCA, but also shortened the production cycle.
The study was published in ACS Synthetic Biology, entitled “Enhancing Cannabichromenic Acid Biosynthesis in Saccharomyces cerevisiae.”
In this study, the biosynthetic pathways of CBCA were precisely reconstructed and multi-layered optimised, making yeast micro-” factories “for efficient CBCA production.
The synthesis process of CBCA involves two key precursors: isoprene pyrophosphate (IPP) and 2, 4-dihydroxy-6-amylbenzoic acid (OA).
By introducing and regulating several key genes, the research team significantly increased the supply of these two precursors, solving the bottleneck problem in CBCA biosynthesis.
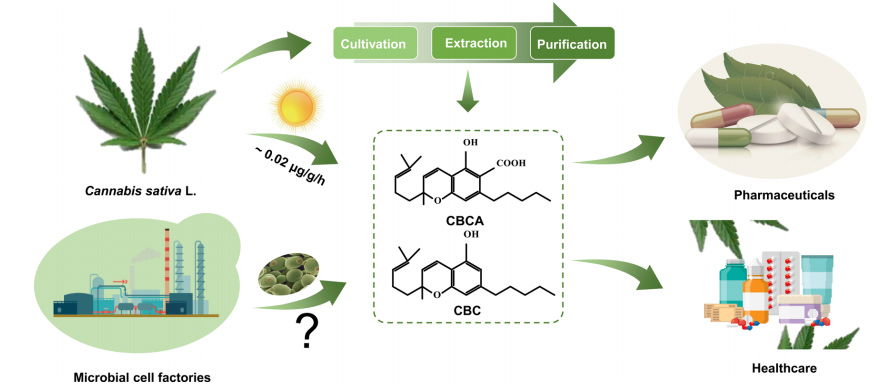
First, to increase the supply of IPP and its isomers, DMAPP, the researchers optimized the yeast’s mevalonate (MVA) pathway, which is the core metabolic pathway for the production of IPP and DMAPP.
They enhanced this pathway by introducing EfmvaE and EfmvaS (genes for acetoacetate-CoA thiolase and HMG-CoA synthetase, respectively) and introducing A mutant ERG20* (F96W/N127W mutation) based on the ERG20 gene. Reduced conversion losses from GPP to FPP, thereby improving GPP availability.
In addition, the team introduced an isopentenol utilization pathway (IUP) that can efficiently generate IPP and DMAPP through only two steps of phosphorylation, which is more simplified and consumes less energy than the 17-step reaction required by the traditional MVA pathway.
Through this strategy, the levels of IPP and DMAPP in yeast significantly increased, and the measured data showed that their content 3.8 times that of the unmodified strain.
At the same time, the synthesis of OA has also effectively improved.
The team constructed a heterogenic pathway that integrates multiple microbial genes, including β-ketothiolase gene (RebktB) of Ralstonia eutropha and butylicum gene (Cacrt) of Clostridium acetobutylicum.
Polyketosynthase genes (CsTKS) and cyclase genes (CsOAC) from cannabis plants introduced.
In addition, they further optimized OA production by adjusting the copy number of key genes such as CsTKS, CsOAC, and CsAAE1, increasing its yield by 32.5% compared to the initial strain.
Next, to achieve the final synthesis of CBCA, the researchers introduced CBCA synthase genes (CBCAS) from the cannabis plant.
However, due to the low activity of this enzyme, the team made CBCAS exhibit higher activity in peroxisomes by adjusting the gene copy number of CBCas in yeast and optimizing its localization.
They also combined with the overexpression of ER helper protein genes, such as ERO1 and PDI1, to solve the bottleneck of enzyme folding and functional expression, thereby greatly improving the efficiency of CBCA production.
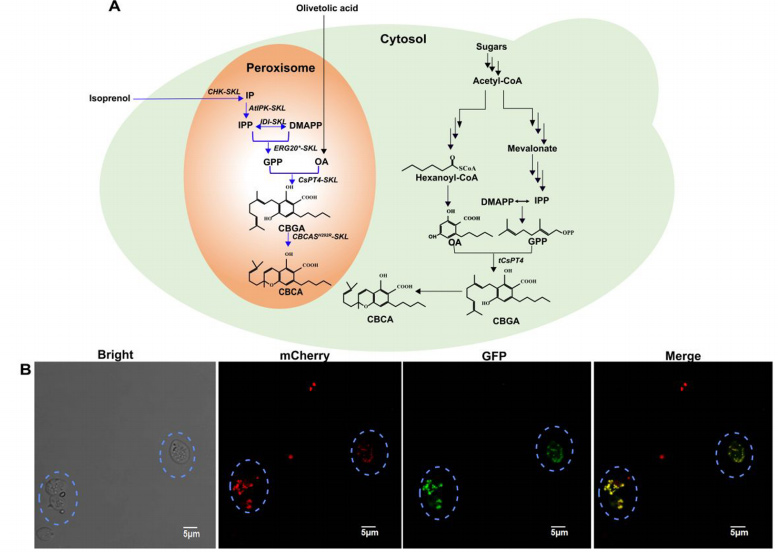
Through careful metabolic engineering, the yeast completed the synthesis from glucose to CBCA in 100 hours, while the traditional cannabis plant’s growth cycle takes about 120 days.
This breakthrough not only significantly shortens the production time, but also reduces the production cost, making the industrial production of CBCA possible.
During fermentation, the optimized yeast strains achieved a CBCA yield of 31.7 μg/L and a CBGA (hemp terpene acid) yield of 12.0 mg in a medium containing glucose and 2, 4-dihydroxy-6-amylbenzoic acid.
These data show that the metabolic potential of yeast has great prospects for development in industrial applications.
What’s more, the study also explored localization of IUP and CBCAS into yeast peroxisomes, a strategy that takes advantage of the isolation properties of subcellular compartments to reduce metabolic by-product production and further increase the production of target molecules.
Through this innovative subcellular engineering, the production efficiency of CBCA has further improved by 26.5%, opening up new research directions for microbial synthesis of complex natural products.
The research of Kang Wei and Xue Chuang’s team demonstrates the powerful potential of synthetic biology in the industrial production of natural products.
Through the precise design of metabolic pathways, the optimization of genetic engineering, and the innovative application of subcellular compartment engineering, yeast has transformed from simple fermenting strains to efficient “factories” of complex molecules.
This approach not only provides a sustainable and efficient solution for the production of CBCA, but also lays the foundation for the microbial synthesis of other cannabinoids and drug-active molecules.
As synthetic biology continues to advance, expect more breakthroughs like this to drive innovation in medicine, biomaterials and energy.
Reference link:
1.Mingming Q, Tian L, Wenqiang Z, et al. Enhancing Cannabichromenic Acid Biosynthesis in Saccharomyces cerevisiae[J].ACS Synthetic Biology Article ASAP,DOI: 10.1021/acssynbio.4c00721.