In the industrial field, nylon 12 has excellent performance, such as low water absorption, low relative density, high wear resistance, flame retardant, etc., and is widely used in 3D printing, automobile manufacturing, oil and gas exploitation, medical equipment, electronic information, auto parts and other fields.
However, the traditional chemical synthesis of nylon 12 has many steps and requires the use of toxic and corrosive raw materials, resulting in high technical thresholds and serious environmental pollution.
Compared with traditional methods, microbial synthesis has significant advantages.
At present, the biological production of nylon 12 monomer mainly involves the conversion of dodecanoic acid (DDA) or its ester to ω-AmDDA or its ester through a multi-enzyme cascade reaction.
The substrates of these studies, DDA and its derivatives, often derived from coconut oil and palm kernel oil, raise significant environmental issues related to deforestation and biodiversity loss;
In addition, the use of edible vegetable oils for industrial grease chemical production has intensified the debate over whether land should be allocated for fuel or food.
Recently, Ye Lidan’s team at Zhejiang University made significant progress in this field, in a study titled “De novo biosynthesis of nylon 12 monomer ω-aminododecanoic acid,” researchers used E. coli bacteria to start from glucose, The efficient synthesis of ω-AmDDA of nylon 12 monomer was successfully realized, which brought a new idea for the sustainable production of nylon 12.
The relevant results were published online in Nature Communications.
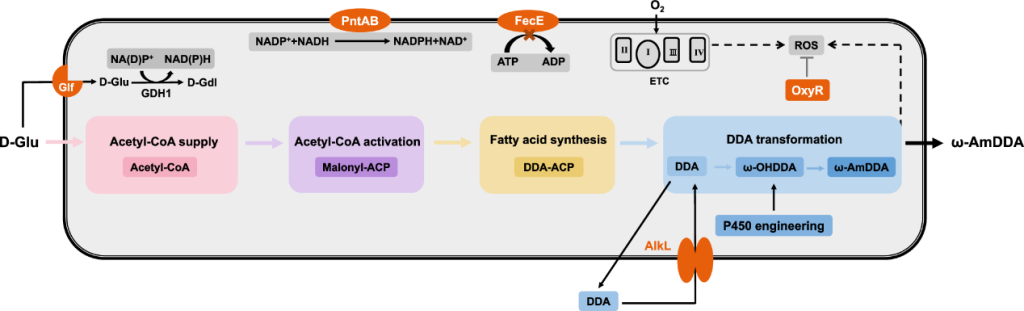
The researchers genetically engineered a complete metabolic pathway for the synthesis of ω-AmDDA in E. coli.
It has previously shown that a high proportion of free DDA can produced in E. coli by introducing thioesterase BTE from California Bay (Umbellularia californica).
If this enzyme can heterogeneously expressed in E. coli along with a multienzyme cascade pathway that converts DDA to ω-AmDDA, selective accumulation of DDA as an intermediate may enable the biosynthesis of ω-AmDDA starting with glucose.
In this study, the researchers introduced thioesterase BTE from California Laurel, which enabled E. coli to selectively synthesize DDA.
DDA converted to ω-AmDDA by multi-enzyme cascade reaction.
This process involves the synergistic action of many enzymes, including hydroxylation catalyzed by P450 enzyme, oxidation reaction of alcohol dehydrogenase and transamination reaction of aminotransferase.
With a series of optimized culture conditions, such as adjusting pH value and concentration of inducer, the yield of ω-AmDDA synthesized by the engineered strain could reach 27.5 mg/L.
Fatty acids (including DDA) may secreted after biosynthesis, leaving the DDA converting enzyme unable to reach the substrate.
Overexpression of the outer membrane protein AlkL from Pseudomonas GPo1 can solve the substrate transfer problem.
When recombinant E. coli strains used for biotransformation using DDA as substrate, overexpression of AlkL can increase the production of ω-AmDDA by 20.3%.
To further optimize yields, the researchers employed a variety of strategies, including:
Modular path engineering
An adequate supply of precursors is essential for efficient production, so enhancing strain synthesis of DDA could further improve the biosynthesis of ω-AmDDA.
According to the central metabolic pathway, the synthesis pathway of DDA divided into three modules:
The upstream glycolysis module, acetyl-CoA activation module and acyl-ACP synthesis module optimized and improved to achieve sufficient but not excessive DDA supply, and the ω-AmDDA titer reaches up to 242.8 mg/L.
Cofactor engineering
By regulating the supply of key cofactors such as NADPH and NADH and enhancing the supply of ATP, the final strain produced 324.4 mg/L of ω-AmDDA after 18 hours of shake-flask fermentation.
Engineering to enhance oxidative stress tolerance
The production and accumulation of DDA and its derivatives will destroy the membrane structure, weaken the function of the electron transport chain located on the membrane, and lead to the accumulation of reactive oxygen species (ROS), and excessive ROS will damage the essential cell components such as cell membrane, proteins and nucleic acids, resulting in ROS stress.
By enhancing oxidative stress tolerance, the constructed strain underwent shake-flask fermentation for 18 h, and the ω-AmDDA yield was 370.9 mg/L, and the biomass increased by 64%.
Protein engineering
In the whole cell catalytic and de novo synthesis of ω-AmDDA, the P450 enzyme hydroxylates DDA to form ω-OHDDA.
The combined mutant CYP153Am4-NCP with increased activity of 89% obtained by saturation mutagenesis of the sites of the possible catalytic domains.
In addition, the protein dimerization of CYP153Am4-NCP enhanced by N-terminal fusion of intermolecular affinity enhancing structure, which further improves the hydroxylation activity.
The ω-AmDDA yield of the final strain was 471.5mg/L after 18h culture, which was 54% higher than that of the control strain, and the DDA accumulation decreased from 43.9mg/L to 25.3mg/L.
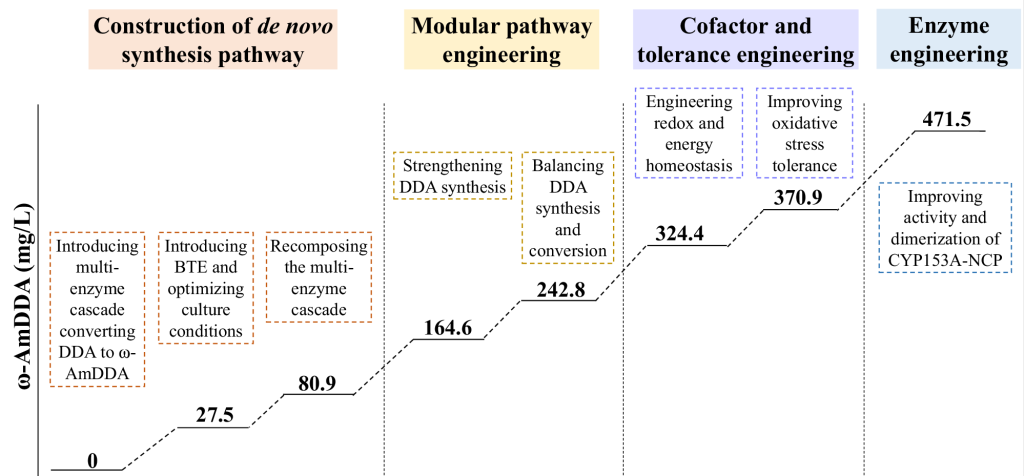
In this study, we developed a multilevel regulatory strategy to achieve efficient de novo biosynthesis of Nylon-12 monomer ω-AmDDA from glucose.
The researchers integrated modular pathway engineering, cofactor engineering, tolerance engineering, and protein engineering to optimize complex synthetic pathways.
By linking endogenous fatty acid metabolism with a heterologous multienzyme cascade that converts DDA to ω-AmDDA, an engineered Escherichia coli strain capable of biosynthesizing ω-AmDDA from glucose constructed.
The researchers strengthened and balanced four pathway modules:
Glycolysis, acetyl-CoA activation, acyl-ACP synthesis and heteroDDA conversion, regulation of NAD(P)H and ATP supply, enhanced oxidative stress tolerance, and rate-limiting P450 designed to re-establish metabolic balance in this cellular factory.
This comprehensive approach could enable the construction of highly efficient ω-AmDDA cell factories, paving the way for sustainable glucose-derived nylon 12 production.
References: https://www.nature.com/articles/s41467-024-55739-0